HUP, EPR, And Bells Theorem
HUP, EPR, and Bell’s Theorem
Abstract
An educational document discussing the Heisenberg Uncertainty Principle, the EPR (Einstein- Podolsky-Rosen) Paradox, and Bell’s Theorem, written for an audience without a background in physics, but with their head still screwed on right.
1 Introduction
Ah, quantum mechanics. A bizarre theory which unfortunately describes our physical world exceed- ingly well. Einstein didn’t get it. Bohr didn’t get it. I don’t get it. And soon, you won’t get it either. As the saying goes, the more you know about quantum mechanics, the less you understand it.
I will be skipping around in terms of topics covered in undergraduate quantum mechanics courses to prepare you for the actual beast, Entanglement.
Entanglement, the property of quantum systems to remain correlated even when separated, is a concept which has transformed from a worrisome byproduct of a thought experiment [1] into a cornerstone of quantum mechanics itself. What is a quantum mechanics? Google is your friend, my dear reader. My time with you is limited„ and I cannot teach you the alphabet to make you read Shakespeare. I can only explain what you directly need to understand this article. Anything else shall be your homework, and if I am feeling kind at the end, I will provide a list of accessible resources on learning quantum mechanics the RIGHT way.
As we dive into the frankly confusing world of entanglement, it is vital that you remember one thing– A quantum particle is described by a wave function, Ψ. This wave function is a solution to the Schrodinger equation.

This is what they mean when they say something is both a particle and a wave; It’s behavior can be described by a special kind of wave equation, which we all know and love as the Schrodinger Wave Equation. But that’s not important right now. I’ll explain more if I need to. We need to get to HUP.
2 Heisenberg’s Uncertainty Principle
Formulated by Werner Heisenberg in 1927, the Uncertainty Principle is an indomitable tenet in the field of quantum mechanics. Its premise is simple. The more precisely a particle’s momentum is determined, the less precisely is its momentum. In one dimension, this can be summarized with the following mathematical statement: ∆x∆p ≤ 2
Here, ∆x is the standard deviation or “spread” of the position x, while ∆p is the standard deviation of the momentum p. As the spread of one quantity decreases, the other must increase in order to maintain the inequality. I will not bother proving the Principle in full, but I have Heisenberg’s original proof in the references.
Is that it?
Ummmmm, no. An important thing to remember about HUP is that it is not exclusive to x and p. HUP applies to any two quantum mechanical operators, A, B, which do not commute with each other i.e. [A, B] = AB − BA = 0. But that’s all mathematical nonsense, Min! What does it really mean?
Fine! I’m only doing this because it will be useful when we get to measurements in the EPR paradox and Bell theorems. In order to understand what “not commuting” means in the physical sense, let’s use our favorites, position and momentum, as an example. In quantum mechanics, xˆ andpˆ are referred to as the position and momentum operators respectively. (Why the little hats? Firstly, they’re cute, and secondly, well, you’ll see.) The whole point of calling them operators is that they act on wave functions. And in the crudest sense possible (please don’t try this at home, folks), hitting an operator on a wave function and taking the expectation value, gives a measurement of the quantum mechanical system.
There is about three semesters of quantum mechanical education I’m waving off right now, but bear with me. When we act the momentum operator on the system, in some sense we extract the momentum. Same thing for position. However, the whole deal about x and p is that they do not commute. So, the order in which you conduct the measurements absolutely does matter. First measuring x and then p would give you a different answer than first measuring p and then x. This is because the very act of measuring a quantum state changes it. That’s right! It changes. This makes all the difference when you consider the standard deviation of a bunch of measurements. If my memory of introductory quantum mechanics serves me right, after about three pages of algebra you arrive at the familiar position-momentum uncertainty principle.
The moral of the story is that the non-commutativity of these operators manifests as a sort of granularity in the accuracy of measurements you can make on a physical system. This granularity is retained between any other kinds of non-commuting measurements you can make!
On second thought, do you really need this? Probably not. But, the algebra of uncertainty principles is a pet project to me. Especially the strangest of them all, the energy-time uncertainty principle. Enough on that! Here’s the main takeaway (other than the actual HUP statement) that you need from this section:
Making a measurement on a state changes its wave function. No exceptions. None. The detached observer is not a reality in the quantum mechanical world.
3 Spin
I realized that the following sections will not make any sense if you don’t at least know what spin is. So, let’s make a short pit-stop at Spin City to learn about this nonsensical physical quantity.
We’re all aware of angular momentum– its the rotational analog of linear momentum (which we talked about the previous section). We all agree that it is a property related to the motion of an object, right? WRONG! Sometime in the 1900s (Seriously, 20th Century Physicists should chill out), it was discovered this angular momentum from motion i.e. “orbital” angular momentum, as it was called in the atomic physics context it was first described, does not account for all the angular momentum of a particle. Long story short, the remaining angular momentum, which is intrinsic to a particle, is now called Spin. Every fundamental particle has a particular value of spin, which, in quantum mechanical jargon, is the eigenvalue of the spin operator.
For understanding the following sections, we really only need to care about spin-1/2 particles, which are lovingly called fermions, and are the building blocks of all ordinary matter. The shining feature of spin-1/2 particles is that their spin can either be +1 or −1 , which is often referred to as spin-up (↑) and spin-down (↓) respectively.
Physically, the up or down comes from whether the measured spin is along the axis it is measured, or opposite to it. Yes, spin is a vector, so it does have three independent components in the three spatial directions, but it is convention to consider the z-component of the spin for calculations and experiments. Any references to up and down in the next sections are along the z-direction.
Oh, and one more thing, spin-0 particles have no intrinsic spin. This will be important when we encounter the EPR Paradox.
4 EPR Paradox
After skipping a whole bunch of most-likely important concepts in the study of quantum mechanics we arrive at the EPR paradox.
The EPR paradox is a thought experiment first described in the groundbreaking paper [1] by Einstein, Podolsky, and Rosen in 1935. Einstein was quite vocally a hater, and the EPR paradox was proposed as evidence that the description of reality provided by quantum mechanics is incomplete. Reality doesn’t care, of course, and the EPR Paradox isn’t really a paradox. In fact, it is the foundation of entanglement– a magnificent, very real feature of reality which spans black holes, quantum computers and even my field of research: Entanglement in elementary particle physics.
In fact, I’m so self-centered that the example we will use to illustrate the EPR paradox is from particle physics. Just kidding, my explanation follows Chapter 12 in Griffiths’ Introduction to Quantum Mechanics, and is a simplified version credited to David Bohm
EPRB Paradox
Suppose a pion (funky particle with spin-0) at rest, decays to an electron and positron which fly off into opposite directions. Since the pion has spin-0, conservation of angular momentum dictates that the electron and positron occupy the following spin configuration.
√(1/2) (|↑↓⟩−|↓↑⟩)
BE NOT AFRAID of the mathematical jumpscare. The fancy bracket |·⟩ is what’s called a “ket”, and is used to denote the state of a quantum system. All the expression says is that either the electron is
spin-up (+1) and the positron is spin-down (−1) or vice-versa, because the total spin of the system 22
must add up to 0. (Since the initial state is spin zero, the system must stay spin-zero even after the decay occurs. That’s what angular momentum conservation is all about.) We don’t know which combination we will get, but it must be one of the above. Measuring the spin of one of the particles will automatically tell us what the spin of the other particle is. This means that the spins of the electron and positron are correlated. In modern terms, such a state is called entangled.
Now, let’s pretend that these particles fly off in opposite directions, until say, they are several light years apart. What would happen if we found the electron and measured its spin to be +1 ? We instantly know that the positron’s spin is −1 . This is obvious. Why are we mad about this?
Naturally, we may think that the electron really was spin-up from the moment it was created and it was only that quantum mechanics did not know until we made a measurement. But by the principles of quantum mechanics, neither particle had a definite spin, until we made a measurement, causing the wave function to “collapse” and instanteously produce the spin of the positron which is lights years away!
The EPR bros were NOT having it. Einstein famously called this phenomenon “spooky action at a distance”. They stated that the quantum mechanical standpoint must be wrong! The electron and positron must have had well-defined spins from their creation, even if quantum mechanics does not know it. Quantum mechanics is not a complete description of reality and there must be some hidden variables which describe a physical system that we do not yet know.
The fundamental assumption guiding the EPR argument is that no information can propagate faster than light. This the principle of locality. In order to appease this, we can say that the wave function collapsed at some finite velocity and is not instantaneous. However, this violates conservation– If we measured the positron spin as well before the information of collapse reached it, there is a 50–50 chance that both particles are spin-up, which means the system has total spin-1. Preposterous! You can mess with anything you want in this universe, but you don’t mess with conservation laws. What do we do now?
Okay, let’s calm down. The theorists may say whatever they want, but experiment doesn’t lie. Experiment tells us that in these cases, spin is perfectly correlated. The wave function collapse is instantaneous. That’s crazy. Call your mom and tell her you want to go home. The EPR Bros are frightening you— Quantum Mechanics is NOT local so it is NOT complete.
...Except. It is. Enter, Bell’s Theorem.
5 Bell’s Theorem
Now, what’s the situation? The EPR gang is not happy. I’m not happy. You’re not happy. Is quantum mechanics wrong? No, silly! EPR said it themselves: they think it’s merely incomplete. So, in order to completely describe a quantum mechanical state, you not only need the wave function Ψ, you also need some unknown, hidden variable λ. Lots of hidden variable theories were proposed after the Einstein-Podolsky-Rosen paper, but none of them ever gained traction. It was still a respectable area of study until 1964, when J.S. Bell proved that any local (Remember locality from the last section?) hidden variable theory is incompatible with quantum mechanics.
I’ll spare you the details of Bell’s work, dear reader. One thought experiment in an essay is gruesome enough. (It is also getting quite late and I still didn’t code my calculations. I have spent far too much time on this already.)
Bell’s proof involves the wonderful use of probability, and the barest assumptions that can be made about local hidden variable theories. Basically, in any local hidden variable theory, the probabilities of various outcomes are related by what’s known as a Bell inequality. If EPR’s conjecture is right, and there really are hidden variables we don’t know about, then any physical system must obey its Bell inequality.
Except, there have been various experiments since the 1960s confirming that Bell’s inequality is indeed violated. This came as a rude shock to scientists as it is not fun to learn that reality is very much nonlocal. It was all fun and games when this was all merely a mathematical artifact, but nonlocality felt like a gateway drug to a much grimmer violation.
Causality
Bell inequality violations, no matter how surprising, are merely wonderful correlations between two sets of otherwise random data. Sure, the measurement of the spin of the electron affects the positron, but it does not cause it in any meaningful way. The person measuring the electron spin cannot use this collapse of the wave function to send a message to the person with the positron, since they don’t control the outcome of the experiment. They can decide whether to measure the electron at all, but the other person only has access to the positron’s spin and cannot tell whether the electron has been measured or not.
Phew! This sort of nonlocal influence does not transmit any energy or information, so it is exempt from the speed of light. Meanwhile, causal influences, those which do transmit information or energy, cannot travel faster than light. According to special relativity, if this was possible then, there are reference frames in which information can propagate backwards through time. And that, my dear reader, is what we call a big nono. Since the EPR paradox does not imply that causality is violated, we can lie uncomfortably on our bed of nonlocal but causal theory of quantum mechanics.
So rest easy, quantum mechanics is weird, but safe. Entanglement is not a fairytale, but also not the boogeyman. It’s probably more scared of you than you of it. Just give it some time. More answers will follow.
What Do I Do Now?
So, you want to know more? Or curl up in a ball and never think about this again? Either is fine. I won’t judge. If your answer is the former, here are some resources to guide you through the thickets of quantum mechanics.
PopSci Sources
1. IDTIMWYTIM: Heisenberg Uncertainty Principle 2. Why did Quantum Entanglement Win the Nobel Prize in Physics? 3. Bell’s Theorem: The Quantum Venn Diagram Paradox
Surely, you’ll get more out of these wonderful science Youtubers than you did from me yapping for four pages. There are a bunch more probably, but you’ll have to find them yourself.
Academic Sources
1. An Introduction to Quantum Mechanics, D.J. Griffiths.
Of course, there are other quantum mechanics textbooks that I like much more than this one. But, this is the least daunting, so I’ll leave it here.
Don’t forget to like and subscribe for more silly academic style papers.
References
[1] A. Einstein, B. Podolsky and N. Rosen, “Can quantum mechanical description of physical reality be considered complete”? Phys. Rev. 47, 777–780 (1935) doi:10.1103/PhysRev.47.777
[2] Heisenberg, W. “Über den anschaulichen Inhalt der quantentheoretischen Kinematik und Mechanik”. Z. Physik 43, 172–-198 (1927). https://doi.org/10.1007/BF01397280
[3] D.J. Griffiths, D.F. Schroeter, “Introduction to Quantum Mechanics, Third Edition” Cambridge University Press (2018) 978–1–107–18963–8,
-
emotionallyconstipated liked this · 5 months ago
-
denimdaydreams liked this · 6 months ago
-
realcyhsy liked this · 6 months ago
-
bmartynec liked this · 6 months ago
-
snakeshae liked this · 6 months ago
-
stavrakas liked this · 6 months ago
-
higgsbosom reblogged this · 6 months ago
-
timetravellingkitty liked this · 6 months ago
-
starznicky liked this · 6 months ago
-
higgsbosom liked this · 6 months ago
-
epidermish reblogged this · 6 months ago
-
meto4 liked this · 6 months ago
-
mrmoonlight78-best-of-beauty liked this · 6 months ago
-
a-dash-in-the-middle liked this · 6 months ago
-
monster-fuzz liked this · 6 months ago
-
princepsmathematics liked this · 6 months ago
-
boekenwuurm liked this · 6 months ago
-
lurking-in-the-darkside liked this · 6 months ago
-
epdrmsh liked this · 6 months ago
-
pepchekoyurate liked this · 6 months ago
-
sincerelycheesecake liked this · 6 months ago
-
dustspeck742 liked this · 6 months ago
-
studyaxis reblogged this · 6 months ago
-
thiscouldntbeworse liked this · 6 months ago
-
zero-body liked this · 6 months ago
-
dondizzzzzle reblogged this · 6 months ago
-
dondizzzzzle liked this · 6 months ago
-
honeynebula liked this · 6 months ago
-
chanthra liked this · 6 months ago
-
migatosabefisica liked this · 6 months ago
-
hyacinth-dancing-in-rain liked this · 6 months ago
-
saintcarrionn liked this · 6 months ago
-
lexinotsoft liked this · 6 months ago
-
kwarrtz liked this · 6 months ago
-
spacetimewithstuartgary reblogged this · 6 months ago
-
spacetimewithstuartgary liked this · 6 months ago
-
zabrien liked this · 6 months ago
-
beautyofsoul reblogged this · 6 months ago
-
xxnylon-blog liked this · 6 months ago
-
iretonmasade liked this · 6 months ago
-
rulerfraggot liked this · 6 months ago
-
incogneeto liked this · 6 months ago
More Posts from Partridge-in-a-pear-tree
Oh shit I just realized I can post the "Gaussian Blur Wizard That Gaussian Blurs You" here

in the 1940s the word “boner” used to mean “huge mistake” and it still pretty much means that
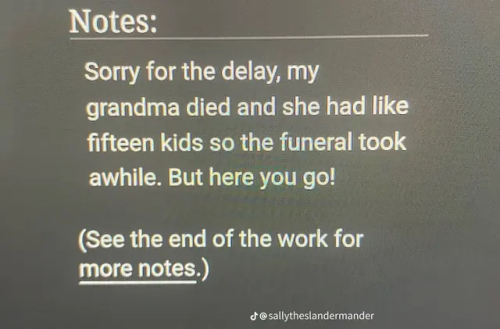
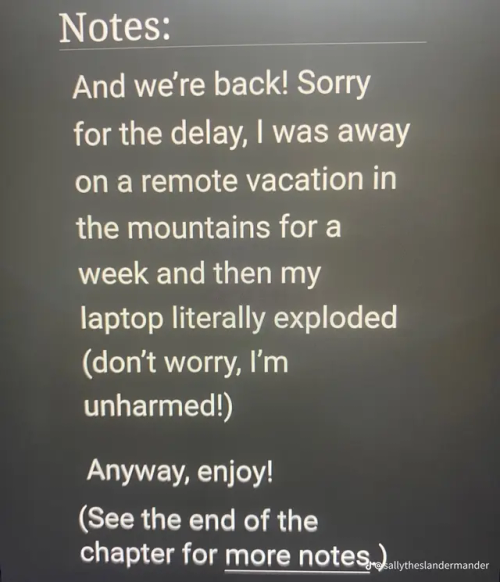
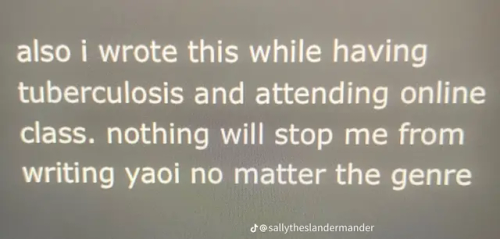
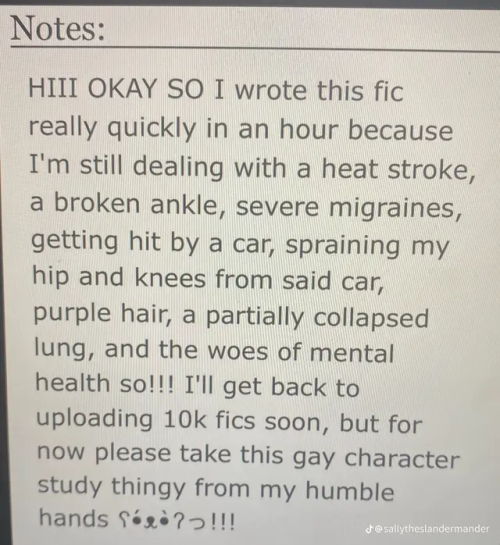

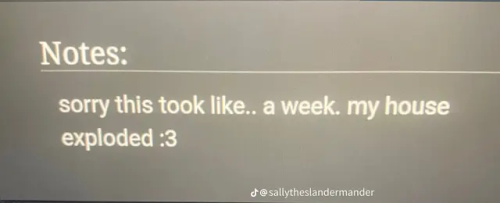
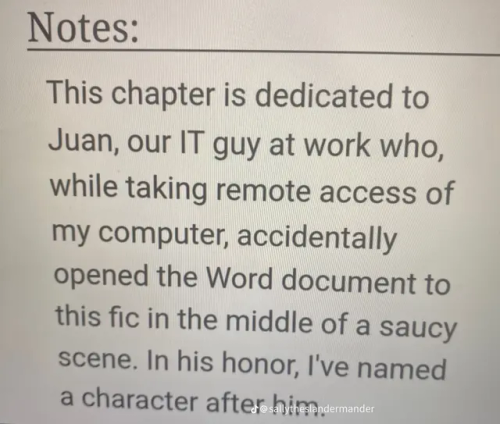
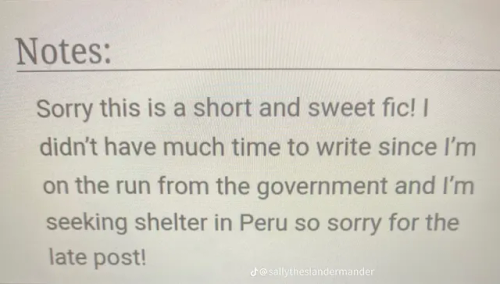
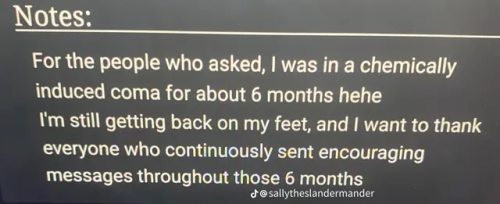
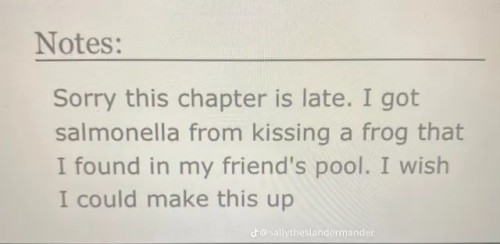
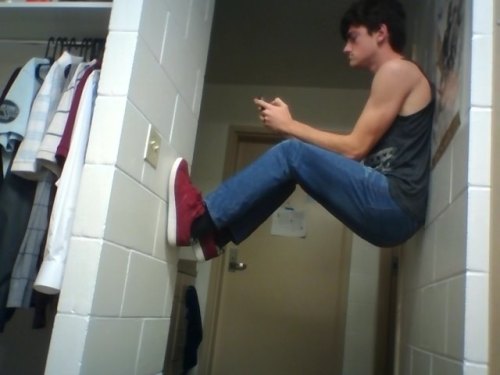
chillin on a Saturday night